Pilots 2023-24
The Delaware Center for Musculoskeletal Research Pilot Awards offer needed assistance to early-career investigators on the path to research independence.
Alvin W. Su, MD, PhD
Sports Medicine Surgeon; Pediatric Orthopedic Surgeon
Nemours Children’s Health, Delaware Valley
Assistant Professor of Orthopaedic Surgery, Thomas Jefferson University
Assistant Professor of Biomedical Engineering, University of Delaware
Profile
Thematic area: translational orthopedic research
Project title: Effect of Weight-bearing and Range of Motion on Repaired Meniscus Mechanics
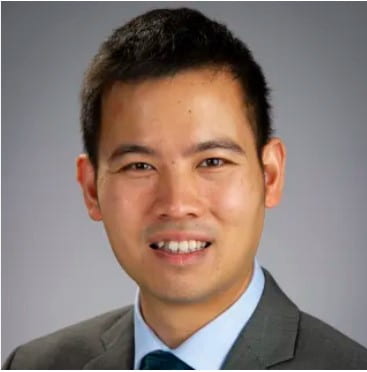
Summary: Surgical repair of meniscus tears is regularly performed and highly successful, however, there is no consensus on the post operative rehabilitation protocol, including the amount of early weight bearing and range of motion. Our long-term goal is to provide evidence-based guidelines for post-operative rehabilitation following meniscus repair. The objective of this proposal is to quantify meniscus deformation and repair integrity using MRI and a loading device that replicates rehabilitation protocols. We hypothesize that early weight bearing with full range of motion for daily life (0° to 90° flexion) does not compromise the integrity of the repair. Using cadaveric knees, we will quantify meniscus mechanics and repair integrity after suture repair of a vertical longitudinal tear with applied one body weight and 0° to 90° flexion using a MRI-compatible knee loading device. These studies in cadaver knees will provide critical pilot data for future work to quantify meniscus mechanics in healthy volunteers and both meniscus mechanics and repair integrity in suture-repair patients. This study will establish in vivo quantification of meniscus mechanics due to loading in post-operative rehabilitation. Further, it will set the stage for Dr. Su to pursue a surgeon-scientist career and establish a new collaboration between Dr. Su and Dr. Elliott.
Pilots 2022-23
Emily Day, PhD
Associate Professor
Biomedical Engineering
Material Science & Engineering
University of Delaware
Profile
Thematic area: Nanomedicine and osteoporosis
Project title: Membrane-wrapped nanoparticles for targeted cargo delivery to mesenchymal stem cells

Summary: This project seeks to develop a biologically inspired nanomedicine that enables safe and effective treatment of osteoporosis, a devastating disease that affects over 10 million Americans annually. The proposed technology consists of cargo-loaded polymer nanoparticles that are wrapped with membranes derived from mesenchymal stem cells (MSCs), which are the precursors to bone-forming osteoblasts. We postulate that the unique proteins expressed on the MSC membrane surface will enable the MSC membrane-wrapped nanoparticles (MWNPs) to selectively bind and deliver cargo to MSCs both in vitro and in vivo. By manipulating MSCs through drug or nucleic acid delivery, it may be possible to enhance osteoblast activity and thereby improve bone density and decrease the risk of fractures. Towards this goal, we propose to (1) evaluate the ability of MSC MWNPs to bind and enter MSCs in vitro without inducing cellular toxicity and (2) demonstrate that MSC MWNPs, but not non-targeted polymer NPs, preferentially interact with MSCs versus hematopoietic stem cells (HSCs) in vitro. Validating the MSC MWNPs can effectively target MSCs in vitro will support future in vivo evaluation and development of this platform for treatment of osteoporosis and other bone diseases.
X. Lucas Lu, PhD
Associate Professor
Mechanical Engineering
University of Delaware
Profile
Thematic area: Cartilage biomechanics
Project title: Resveratrol for the Protection of Osteocyte Mechanotransduction
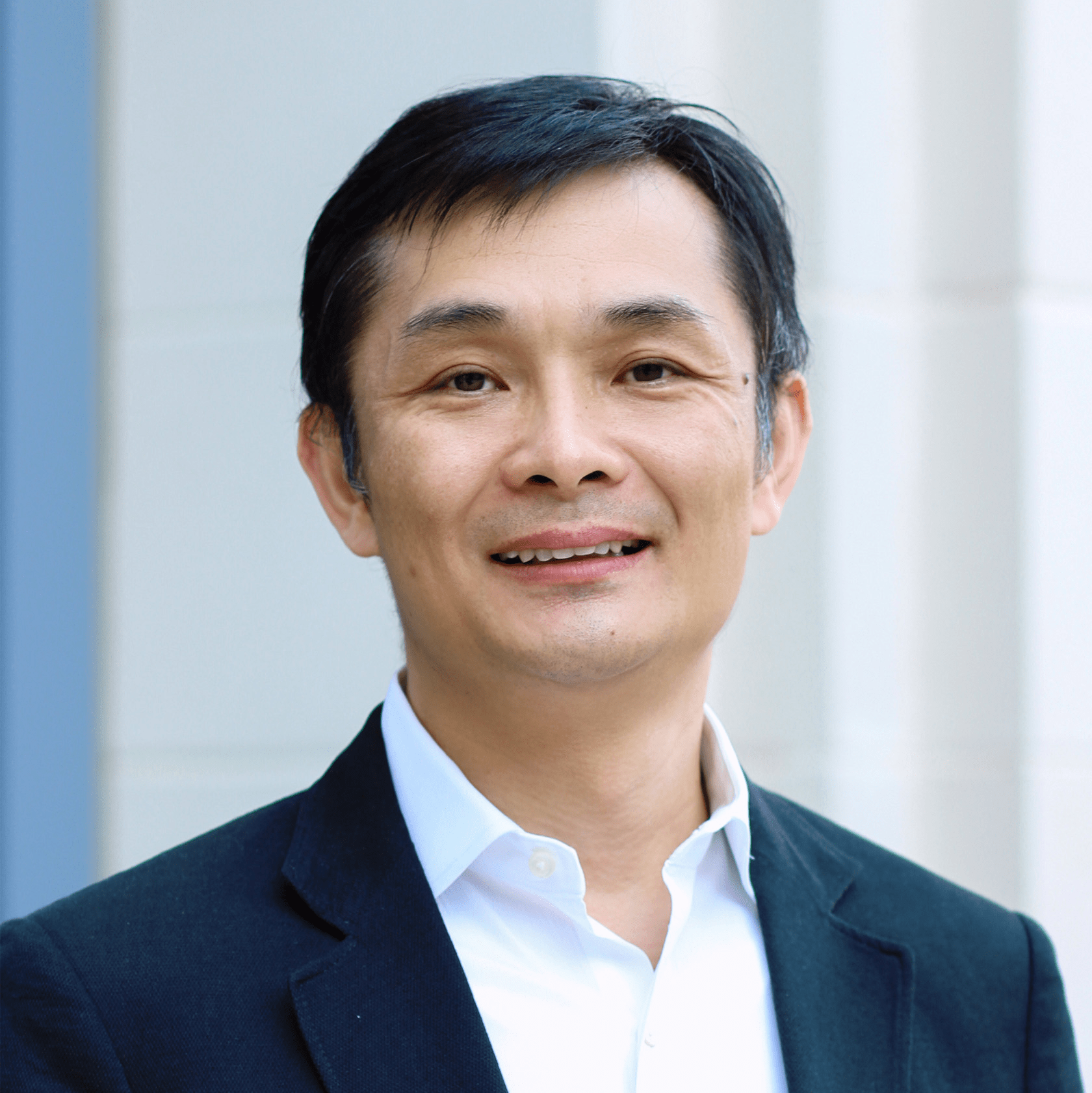
Summary: This project aims to study the potential of using resveratrol as a food supplement to reduce postmenopausal bone loss. Resveratrol is a natural antioxidant presented in the skin of grapes, red wine, and berries. Many studies in cancer and anti-aging research have revealed the health beneficial effects of resveratrol. A recent clinical trial showed that resveratrol can slow down bone loss in postmenopausal women. We have found that resveratrol can attach on collagen molecules and protect collagen fibrils from enzymatic degradation. Resveratrol can also inhibit the activities of matrix metalloproteinases. Based on these finding, we propose to test a new bone-protective mechanism of resveratrol, i.e., resveratrol can protect the integrity of the osteocyte pericellular matrix post menopause by simultaneously strengthening the collagen fibrils and inhibiting collagen degradation. We will first test the hypothesis using an ovariectomized mouse model. The mice received resveratrol in food are expected to have higher density of PCM tethers and more robust responses under loading. The unique protective mechanisms of resveratrol will then be defined using cell and bone explant culture models. MMP activity assays will be performed to determine the inhibition efficacy of resveratrol on various MMPs. The outcome of this project could motivate resveratrol consumption in the population who are suffering from or at a high risk of bone loss. The project aligns with the DCMR missions that include the “identifying potential therapeutic intervention” to improve skeletal health.
Pilots 2021-22
Charles Dhong, PhD
Assistant Professor
Material Science & Engineering
Biomedical Engineering
University of Delaware
Profile
Thematic area: Osteoarthritis & Diagnosis
Project title: Resolving chondrocyte stiffening from matrix stiffening in osteoarthritis models
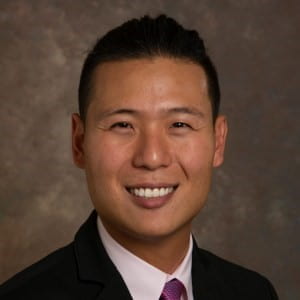
Summary: Although multiple mechanisms have been identified as a target for osteoarthritis, current therapies are ultimately not effective at reversing osteoarthritis and no treatment option exists. To provide new treatment strategies, our long-term goals are to provide a comprehensive timeline of the early stages of osteoarthritis, which has been difficult because osteoarthritis originates from closely associated changes between the collagenous extracellular matrix and chondrocytes. This pilot project will provide a reference of mechanical changes in cartilage undergoing simulated conditions which mimic early stages of osteoarthritis. Crucially, we will decouple key processes in the extracellular matrix from those originating within the chondrocytes in pre-osteoarthritis. Our approach uses in situ, highly sensitive strain sensors placed onto cartilage explants undergoing simulated osteoarthritis. In separate experiments, simulated osteoarthritis will target either extracellular matrix degradation or aberrant chondrocyte physiology. The mechanical stiffness of cartilage is a powerful biomarker of key stages of osteoarthritis because it synthesizes contributions from multiple pathways, but, by the same reason, it is historically challenging to attribute changes in stiffness to specific processes. Due to our time-resolved in situ measurements, high sensitivity, and matrix/filler analysis, we will be able to attribute mechanical stiffness primarily to the extracellular matrix or chondrocytes. Characteristic mechanical changes in the extracellular matrix will be separated from changes within chondrocytes in two aims. In the first aim, the extracellular matrix will be targeted by simulating stages of osteoarthritis which primarily degrade the extracellular matrix, including degradation from cellular processes or mechanical injury. In the second aim, characteristic mechanical changes attributable to the chondrocytes—proliferation, dedifferentiation, and inflammatory responses, will be simulated through soluble factors. While we expect crosstalk between the chondrocytes and extracellular matrix, our time-resolved measurements will help classify mechanical changes to a primary source. The significance of a more unified timeline of osteoarthritis progression may help redeploy or combine existing strategies to provide new treatments.
Christopher Price, PhD
Associate Professor
Biomedical Engineering
University of Delaware
Profile
Thematic area: Articular cartilage and bone
Project title: Chemogenetic Approaches for Promoting Chondrogenesis and Cartilage Tissue Engineering

Summary: As a result of articular cartilage’s limited ability to spontaneously regenerate/heal in vivo, focus has been placed on the use of cell-based tissue-engineering/regeneration strategies to aid cartilage repair. However, ‘native-tissue-like’ repair of articular cartilage represents a major, and yet unrealized, clinical challenge, in part due to an incomplete understanding of the (mechano) biology of articular cartilage development and the presence of technical hurdles to scale-up. Nonetheless, one mechanism conclusively tied to chondrogenesis and chondrocyte physiology is intracellular calcium signaling ([Ca2+]i). Calcium is a ubiquitous second messenger crucial for regulating the proliferation, differentiation, homeostasis, and matrix metabolism of chondrocytes and their precursors (e.g., mesenchymal stem cells [MSCs]). Mature chondrocytes and MSCs exhibit robust spontaneous [Ca2+]i signaling, which have been linked to enhanced chondrogenic and increased matrix synthesis (anabolic) outcomes. [Ca2+]i activation is also the earliest, and an obligate response of chondrocytes and MSCs in their adaptation to the necessary mechanical stimulation required for articular cartilage development and health. However, controversy remains regarding the primary drivers of chondrocytes [Ca2+]i signaling. Nonetheless, mechanical loading is routinely utilized as an adjuvant to improve/accelerate cartilage tissue engineering; however, physically driving construct maturation through mechanical loading is not without limitations. Alternatively, recent studies have demonstrated the utility of non-physical manipulation of chondrocyte ‘mechanosensory’ pathways in promoting engineered cartilage development and maturation. Indeed, exogenous manipulation of [Ca2+]i through targeting native TRPV4 calcium channels enhances MSC chondrogenesis, and matrix deposition in and maturation of engineered cartilage constructs. Building upon such findings, the PI proposes the use of a novel chemogenetic strategy, the use of the engineered designer receptors exclusively activated by designer drugs [DREADDs] to: i) demonstrate the capacity for synthetic activation of [Ca2+]i-regulating Gaq- & Gas-coupled DREADD-GPCR cascades to control chondrogenesis, and chondrocyte (re)differentiation, physiology and matrix metabolism in both primary chondrocytes and their MSC precursors, and ii) establish the efficacy of synthetically driven DREADD-mediated Ga-protein signaling for enhancing the development of MSC and/or primary chondrocyte-derived engineered 3D cartilage tissues. Ultimately, this work will introduce a simple, yet transformative methodology for precisely studying the role of GPCR-driven [Ca2+]i signaling in chondrogenesis and cartilage physiology while providing a novel tool for enhancing engineered cartilage maturation, and improving cartilage tissue engineering for joint repair and regeneration.