Pilot Projects 2020-21
Charles Dhong, PhD
Assistant Professor
Material Science & Biomedical Engineering
University of Delaware
Thematic area: Osteoarthritis & Diagnosis
Project title: Resolving chondrocyte stiffening from matrix stiffening in osteoarthritis models
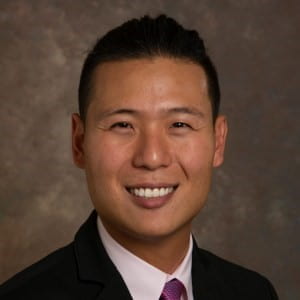
Summary: Although multiple mechanisms have been identified as a target for osteoarthritis, current therapies are ultimately not effective at reversing osteoarthritis and no treatment option exists. To provide new treatment strategies, our long-term goals are to provide a comprehensive timeline of the early stages of osteoarthritis, which has been difficult because osteoarthritis originates from closely associated changes between the collagenous extracellular matrix and chondrocytes. This pilot project will provide a reference of mechanical changes in cartilage undergoing simulated conditions which mimic early stages of osteoarthritis. Crucially, we will decouple key processes in the extracellular matrix from those originating within the chondrocytes in pre-osteoarthritis. Our approach uses in situ, highly sensitive strain sensors placed onto cartilage explants undergoing simulated osteoarthritis. In separate experiments, simulated osteoarthritis will target either extracellular matrix degradation or aberrant chondrocyte physiology. The mechanical stiffness of cartilage is a powerful biomarker of key stages of osteoarthritis because it synthesizes contributions from multiple pathways, but, by the same reason, it is historically challenging to attribute changes in stiffness to specific processes. Due to our time-resolved in situ measurements, high sensitivity, and matrix/filler analysis, we will be able to attribute mechanical stiffness primarily to the extracellular matrix or chondrocytes. Characteristic mechanical changes in the extracellular matrix will be separated from changes within chondrocytes in two aims. In the first aim, the extracellular matrix will be targeted by simulating stages of osteoarthritis which primarily degrade the extracellular matrix, including degradation from cellular processes or mechanical injury. In the second aim, characteristic mechanical changes attributable to the chondrocytes—proliferation, dedifferentiation, and inflammatory responses, will be simulated through soluble factors. While we expect crosstalk between the chondrocytes and extracellular matrix, our time-resolved measurements will help classify mechanical changes to a primary source. The significance of a more unified timeline of osteoarthritis progression may help redeploy or combine existing strategies to provide new treatments.
Christopher Price, PhD
Associate Professor
Biomedical Engineering
University of Delaware
Thematic area: Articular cartilage and bone
Project title: Chemogenetic Approaches for Promoting Chondrogenesis and Cartilage Tissue Engineering

Summary: As a result of articular cartilage’s limited ability to spontaneously regenerate/heal in vivo, focus has been placed on the use of cell-based tissue-engineering/regeneration strategies to aid cartilage repair. However, ‘native-tissue-like’ repair of articular cartilage represents a major, and yet unrealized, clinical challenge, in part due to an incomplete understanding of the (mechano) biology of articular cartilage development and the presence of technical hurdles to scale-up. Nonetheless, one mechanism conclusively tied to chondrogenesis and chondrocyte physiology is intracellular calcium signaling ([Ca2+]i). Calcium is a ubiquitous second messenger crucial for regulating the proliferation, differentiation, homeostasis, and matrix metabolism of chondrocytes and their precursors (e.g., mesenchymal stem cells [MSCs]). Mature chondrocytes and MSCs exhibit robust spontaneous [Ca2+]i signaling, which have been linked to enhanced chondrogenic and increased matrix synthesis (anabolic) outcomes. [Ca2+]i activation is also the earliest, and an obligate response of chondrocytes and MSCs in their adaptation to the necessary mechanical stimulation required for articular cartilage development and health. However, controversy remains regarding the primary drivers of chondrocytes [Ca2+]i signaling. Nonetheless, mechanical loading is routinely utilized as an adjuvant to improve/accelerate cartilage tissue engineering; however, physically driving construct maturation through mechanical loading is not without limitations. Alternatively, recent studies have demonstrated the utility of non-physical manipulation of chondrocyte ‘mechanosensory’ pathways in promoting engineered cartilage development and maturation. Indeed, exogenous manipulation of [Ca2+]i through targeting native TRPV4 calcium channels enhances MSC chondrogenesis, and matrix deposition in and maturation of engineered cartilage constructs. Building upon such findings, the PI proposes the use of a novel chemogenetic strategy, the use of the engineered designer receptors exclusively activated by designer drugs [DREADDs] to: i) demonstrate the capacity for synthetic activation of [Ca2+]i-regulating Gaq- & Gas-coupled DREADD-GPCR cascades to control chondrogenesis, and chondrocyte (re)differentiation, physiology and matrix metabolism in both primary chondrocytes and their MSC precursors, and ii) establish the efficacy of synthetically driven DREADD-mediated Ga-protein signaling for enhancing the development of MSC and/or primary chondrocyte-derived engineered 3D cartilage tissues. Ultimately, this work will introduce a simple, yet transformative methodology for precisely studying the role of GPCR-driven [Ca2+]i signaling in chondrogenesis and cartilage physiology while providing a novel tool for enhancing engineered cartilage maturation, and improving cartilage tissue engineering for joint repair and regeneration.